Haruhiko Okumura On Twitter: Letterfix For Mac
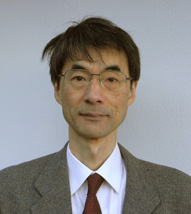
Advertisement If you’ve used a computer for any length of time, you will have no doubt encountered the Blue Screen of Death, or a kernel panic, where the Windows 8 isn't perfect. That said, most blue screens and application crashes aren't Windows' fault. Our tips will help you identify exactly what's wrong with your PC and fix it., costing you all your work. What causes this to happen, and is there anything you can do to prevent it in future? Aff repair station keygen for mac. Let’s take a look. What Is a Kernel Panic and What Causes One? A kernel panic, or its equivalent in the Windows world of a stop error or the (BSOD), happens as the result of an unspecified low level error that an operating system cannot recover from.
The error is regarded as being more serious than a simple crashing app, and potentially damaging to the system or data. As a result the operating system takes the decision to close everything down as a safety measure, and output error codes containing information about what exactly happened. From the user’s perspective, all it means is that one minute you’re working as normal, and the next, your computer is restarting, and you’ve lost everything you’ve done since you last saved. Linux On There are many ways in which you can try Ubuntu. One of them is to install Ubuntu on Windows 10 using one of these methods. There’s also something called a kernel oops, where a serious error occurs that the operating system is able to deal with. The system will continue running, although it may cause instability and even lead to a full kernel panic, shown as a black screen full of code.
Has anyone ever seen someone, be them a Mac or Windows/Linux user, ever say bad things about Apple's Magic Trackpad? Everyone I know who has experience. Tweet with a location. You can add location information to your Tweets, such as your city or precise location, from the web and via third-party applications. The medium was removed and the pellet was resuspended in 1 ml of 100% methanol at 4°C for 10 minutes to fix the cells. The pellet was washed in 3 ml of PBS and resuspended in 1 ml of PBS containing 1% bovine serum albumin (BSA) and 0.1% serum for 1 hour at room temperature.
A kernel panic on Linux is not always preceded by a kernel oops. The causes of a kernel panic or BSOD are many and varied, and they can be hardware or software-related. Common causes include things like faulty RAM or malfunctioning peripherals, drivers or software plugins, or even badly written programs. What To Do When You Get One When you experience a kernel panic or BSOD, there’s not a lot you can do about it other than restart your computer and get back to work.
The kernel panic generates a log containing data that can help an engineer decipher what exactly has happened. Upon restarting, and depending on your operating system, you should be given the option to send this to the OS developer. You won’t get personalised support for the issue, but it will help them prevent kernel panics in future versions of the operating system.
It’s important to remember that a kernel panic is only a sign that an error occurred, not that there’s anything fundamentally wrong with your PC. Everyone will get them from time to time, and you can happily forget about it. However, if you start to see them on a more regular basis — such as every couple of weeks — then you should try and identify what is causing them. Troubleshooting A Kernel Panic Each time a kernel panic occurs, a log will be created containing information about what happened. It’s mostly incomprehensible to the regular user, although a scan of the data can sometimes reveal a particular app that was at fault. To view the log on Windows, you may need to download and install the. Mostly, though, you’ll just need to investigate some common causes to see if they are to blame.
Hardware Issues When looking for possible causes of your kernel panics, the key is to try and identify any changes you’ve made to your system recently. Undoing these changes, and then re-enabling them one at a time, helps you to isolate a possible cause. Check the RAM: If you have Is your laptop old, slow, and has the hardware never been upgraded? Working on a slow computer can be a real drag.
Before you buy a completely new one, however, you should consider ways to. In your computer, the first step is to check that it is seated properly. If the problem persists, remove the RAM you added. If that solves it, the RAM may be faulty, so contact your retailer for an exchange. Detach Peripherals: It isn’t just large add-ons like scanners and printers that can cause potential problems. A kernel panic can be caused by something as benign as a It's well known that USB flash drives are supposed to be ejected before removing them.
But do you really still need to do this? What happens if you don't?
Once you are confident that a particular device isn’t at fault, you can reconnect it. Check for Disk Errors: Run the Have you ever had a drive failure? Sadly, there’s no panacea for preventing hardware damage. But monitoring your HDD or SSD, to act when their death becomes likely, is a first step. Built into your computer’s operating system to ensure that disk errors are not causing your kernel panics.
If the computer crashes as soon as it boots, you will need to either boot into the Recovery partition (usually F10 on Windows and Command + R on Mac; for Linux it depends on the distro being used) or Want to install Ubuntu but don't have a spare blank DVD? After downloadin the ISO file, you can use a highly-reusable USB flash drive to get the job done.
Haruhiko Okumura On Twitter Letterbox For Mac
To carry out these diagnostic tasks. Software Issues If software is the cause of your kernel panic or Blue Screen of Death, you will tend to pick on which program it is over a period of time. Sometimes, though, applications running in the background, such as security software, may be the cause. To diagnose software problems, In Safe Mode, you can troubleshoot and fix Windows issues. When you enter Safe Mode, Windows loads a minimal environment that ensures a stable system. On Mac or Windows. This loads only the core elements of the operating system.
Do this on Windows by holding F8 when you restart, and on Mac by holding the Shift key after you hear the startup chime. Linux doesn’t have a safe mode as such, only the recovery partition. Check Software and Startup Items: Check all your software is up to date, and also look at which programs are launching on boot. Disable any that you installed shortly before you started to experience kernel panics, then re-enable them in turn. Update the System: Download and install the latest updates for your operating system, as well as drivers for your hardware on Windows. If you’re testing a beta or preview versions of your operating system, these may not be stable so may be a cause of the problem.
Use System Restore: If you make a lot of changes to your system, consider using System Restore or Time Machine to roll back to a time before the kernel panics occurred. Can You Avoid Them in the Future?
Kernel panics and the Blue Screen of Death are pretty rare. You will inevitably experience them from time to time, but they aren’t usually indicative of a wider problem. If you do start to see them on a regular basis, it’s almost always a direct result of a recent change you have made to your system. As such, they’re often relatively easy to diagnose and deal with. There’s no way to avoid kernel panics, but so long as you’re prepared should you ever need to deal with them, there’s no need to worry either. What are your experiences with the dreaded Blue Screen of Death? Have you found any hardware or software that has caused a kernel panic on your Mac?
Let us know in the comments. Image credits: Lots of BSOD via, BSOD via, Mac kernel panic via, Kernel panic Linux via, Macbook RAM via, Peripherals via Explore more about:.
1 Interseismic deformation in Japan results from the combined effects of tectonic processes including rotation of crustal blocks and the earthquake cycle process of elastic strain accumulation about upper plate faults and subduction zone interfaces. We use spherical linear block theory constrained by geodetic observations from densely spaced Global Positioning System (GPS) stations to estimate plate motions, fault slip rates, and spatially variable interplate coupling on the Japan‐Kuril, Sagami, and Nankai subduction zones. The reference model developed in this paper consists of 20 blocks, produces a mean residual velocity magnitude of 1.84 mm/yr at 950 stations, and accounts for 96% of the observed interseismic deformation signal. We estimate fault slip rates in excess of 15 mm/yr along the Niigata‐Kobe Tectonic Zone and Itoigawa‐Shizuoka Tectonic Line through central Japan, confirming their hypothesized roles as major tectonic boundaries.
Oblique convergence across the Nankai Trough is partitioned, with 3/4 of the ∼30 mm/yr of trench‐parallel motion accommodated by strike‐slip motion on the subduction interface and the remaining 1/4 accommodated by right‐lateral slip on the Median Tectonic Line. In contrast, our models suggest negligible slip partitioning in eastern Hokkaido, where oblique slip on the Japan‐Kuril subduction interface accommodates all of the trench‐parallel component of relative plate motion.
Inferred spatial variations in the rake and magnitude of slip deficit on subduction zone interfaces reflect the influences of megathrust geometry and earthquake cycle processes such as enhanced elastic strain accumulation about seismic asperities and coseismic sense fault motion indicative of silent slip events or afterslip following large earthquakes. Introduction 2 Over the last two decades, Global Positioning System (GPS) measurements of crustal motion have provided unprecedented insight into tectonic processes including plate motions, fault system activity, and the earthquake cycle e.g.,;. Modern wide aperture GPS networks comprise hundreds of continuously recording stations capable of tracking spatial and temporal variations in surface motions across entire plate boundary zones, providing insight into the underlying tectonic forces that drive them. The GEONET network of Japan is a type example of such an array, consisting of 1224 GPS stations spaced ∼20 km apart recording daily position estimates with time series durations up to 15 years. The long recording period and dense station spacing make these data ideal for understanding active plate boundary processes and, in particular, the interactions between subduction zones and crustal faults in and around Japan. Plate Tectonic Setting Models 3 The plate tectonic setting of Japan is a debated topic, with proposed models differing in the number, names, sizes, and interactions of tectonic plates e.g.,;;,. This ambiguity stems primarily from difficulty in precisely determining the boundary between the North American (NAM) and Eurasian (EU) plates in northeast Asia , and references therein.
All models are similar in that the Pacific Plate (PAC) subducts beneath central northern Honshu and Hokkaido at the Japan‐Kuril Trench, and the Philippine Sea Plate (PS) subducts obliquely beneath southwestern Honshu and Shikoku along the Nankai Trough, beneath central Honshu but above PAC at the Sagami Trough, and beneath Kyushu and the Ryukyu Islands. Some models suggest that northern Honshu and Hokkaido belong to NAM , while others e.g.place the boundary between NAM and EU through Hokkaido, meaning that most of Japan is part of EU.
Subdivision of NAM and EU around Japan into several smaller plates forms the basis of additional plate tectonic models. The Okhotsk Plate (OK) encompasses much of the Sea of Okhotsk, with its eastern boundary defined by the Japan‐Kuril Trench and the western boundary striking roughly parallel to the long axis of Sakhalin Island. The western boundary of OK near Japan has been proposed to cross Hokkaido or, alternatively, follow the eastern edge of the Sea of Japan and through central Honshu e.g., , with the Sea of Japan portion of the boundary representing a zone of “incipient subduction”. Employs both of these boundaries in proposing a new microplate comprising northern Honshu and southwest Hokkaido. The Amurian Plate (AM) has been subdivided from EU and lies between the Baikal Rift Zone in Russia and OK (or NAM ) and, based on the analysis of, AM is beginning to subduct beneath OK (or NAM) at the incipient subduction zone.
This plate boundary has been highlighted by the occurrence of several moderate to large earthquakes, including the 2007 M w = 6.8 Niigata Prefecture reverse faulting event. Suggest that much of Japan is part of AM, but the eastern part of Hokkaido belongs to OK. They argue that much of northern Honshu is part of a “strike‐slip duplex,” with numerous NNW striking right‐lateral faults dividing the island into distinct blocks. The easternmost of these faults extends north from the prominent bend in the Japan‐Kuril subduction zone (41°N) across Hokkaido and Sakhalin Island, consistent with previous interpretations of the AM‐OK boundary e.g.,.
Find that contemporary GPS velocities in northeast Asia favor inclusion of AM and OK as distinct plates with boundaries similar to those proposed previously e.g.,;. Elastic Deformation Signals 4 In addition to rotational motion of tectonic plates, elastic deformation from both offshore subduction zones and the onshore network of crustal faults produces distinct signatures in the geodetic velocity field. Interseismic elastic strain accumulation due to locking at a subduction zone gives rise to a surface velocity field directed approximately in the direction of plate convergence, with speeds that in general decay with distance away from the plate boundary. Where convergence is oblique to the strike of the subduction zone, geodetic velocities show enhanced deflection away from the trench‐perpendicular direction ;. Block Modeling 6 Nominally interseismic GPS velocities record the combined effects of plate motions, permanent deformation, and elastic strain accumulation.
Using linear spherical block theory , we simultaneously estimate the rotation vectors of crustal blocks, kinematically consistent slip rates on block bounding faults, spatially variable fault slip rates on subduction zone interfaces, and strain rate tensor components within deforming blocks, and we focus on the former three contributions in this paper. 7 Through an idealized earthquake cycle, interseismic elastic strain accumulation complements coseismic strain release, so that there is no net elastic strain accumulation in the crust surrounding seismogenic faults e.g.,. In modern block theory, nominally interseismic velocities, v I, are given by the difference between long‐term block motion, v B, homogeneous internal block strain, and the elastic deformation from the coseismic slip deficit (CSD) associated with both kinematically consistent slip rates on the block bounding faults, v CSD, and partially coupled regions, v TDE, described by triangular dislocation elements (TDEs): v I = v B( Ω) − v CSD( Ω) + v TDE( t) + ( ), where the velocity components are functions of block rotation parameters, Ω, TDE slip rate components, t, or intrablock strain tensors,. We use a linear estimator to simultaneously estimate the block rotation vectors Ω est, smoothly varying TDE slip vectors t est, and intrablock strain tensors est using a weighted least squares inversion.
Fault slip rates are calculated by projecting the rotational block motions onto the geometry of the bounding faults, thus ensuring kinematic consistency. Uncertainties on fault slip rates are calculated by the direct linear propagation of data uncertainties while Euler pole location and rotation rate uncertainties are determined by a Monte Carlo propagation of formal rotation vector uncertainties. Smooth variation of t est is enforced by simultaneously minimizing the data misfit and gradient of the TDE slip distribution, carried out by setting the discrete second derivative of TDE slip equal to zero. The weighting matrix used in the inversion includes data and pseudodata variance, the latter of which is expressed by a coefficient β, which controls the extent to which TDE smoothness is enforced e.g.,. A small value of β permits a better fit to the GPS data at the expense of an oscillating, physically unreasonable TDE slip distribution, while a very large β value strongly enforces smoothing such that t est on each TDE approaches a homogeneous value (see discussion in ).
GPS Data 8 The principal goal of this paper is to assess the tectonic processes in Japan that contribute to the geodetically observed interseismic deformation signal. Our block models are constrained by GPS velocities from the GEONET array in Japan along with several other network across east Asia and the Pacific ;;;;;.
We combine these velocities into the nominal stable Eurasian reference frame of by solving for rotation and translation vectors that minimize differential velocities at collocated stations. Nominally interseismic GPS velocity field in Japan derived from GEONET data between 1997 and 2000, expressed in a stable Eurasian reference frame Apel et al., 2006. Velocity magnitude is indicated both by vector length and color. Dashed lines show plate boundaries from Bird 2003. 9 We determine the velocity field in Japan using the GEONET processed daily position estimates (Geographical Survey Institute, ). For each component of the time series, we correct position offsets introduced by antenna changes (marked by abrupt changes on the order of 10–100 m) and then fit an equation comprising a linear trend (velocity component) and an annual periodic signal.
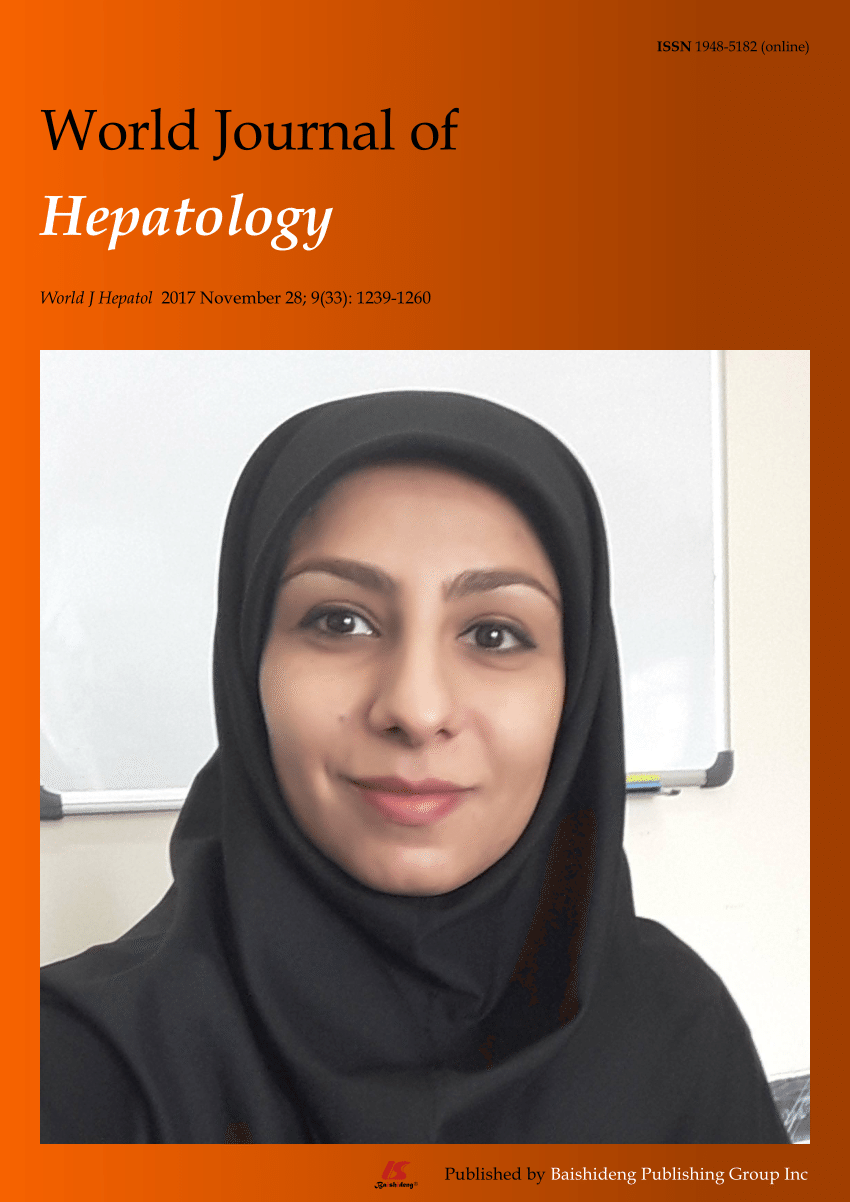
We examine a time series spanning January 1997 to May 2000, limited in temporal extent to avoid contamination of the interseismic signal from coseismic deformation related to several strong to great earthquakes that have occurred throughout the GEONET observation period of 1994 to present. The two M = 6.7 Hyuga‐nada earthquakes of October and December 1996 each induced up to 20–30 mm of coseismic displacement on the east shore of Kyushu. The signal caused by postseismic deformation and a slow slip event following these earthquakes e.g.was not explicitly removed and therefore remains as a contribution to the velocity field in the Kyushu‐Bungo Channel region (“BC” in ). Consideration of the time series ending in 2000 avoids effects from the seismic swarm offshore central Japan in 2000, the great M w = 8.3 Tokachi‐oki earthquake offshore Hokkaido in 2003, and the series of M w ∼ 7.5 earthquakes offshore Sendai between 2003–2005. We account for effects on the velocity field of two episodes (March 1997 and May 1998 , and references therein) of coupled seismic and volcanic activity near the Izu Peninsula (“IP” in ), and two earthquakes that occurred near the southwest Kyushu coast (26 March 1997 and 13 May 1997 ) by solving for a shift in station position following onset of the events.
Comments are closed.